Water and Atmospheric Moisture
Chapter 7
“Have you visited the treasuries of the snow? Have you seen where the hail is made?
Who created a channel for the torrents of rain? Who laid out the path for the lightning?
Who makes the rain fall on barren land, in a desert where no one lives?
Who sends the rain that satisfies the parched ground
and makes the tender grass spring up?
Does the rain have a father? Where does dew come from?
Who is the mother of the ice? Who gives birth to the frost from the heavens?
For the water turns to ice as hard as rock, and the surface of the water freezes.
Do you know the laws of the universe and how God rules the earth?
Can you shout to the clouds and make it rain?
Can you make lightning appear and cause it to strike as you direct it?
Who is wise enough to count all the clouds?
Who can tilt the water jars of heaven, turning the dry dust to clumps of mud?”
Job 38:22-38 (NLT)
**There is a video version of this lecture here: https://youtu.be/fDMoSLG9ih4
**The exam is based on the content in these notes, so please print them off to study from.
I. Water
Water is one of the most fundamental realities of the Earth-atmosphere system. Without water, of course, life on Earth would not be possible.
The hydrologic cycle or water cycle describes the flow of water through the atmosphere (as clouds, fog, precipitation, and water vapour), on the Earth’s surface (runoff, streams, rivers, lakes, glaciers, oceans), and under the surface (groundwater). In this chapter we focus on atmospheric moisture and properties of water in its various states:
- frozen ice (solid state)
- liquid water
- water vapour (gas state)
Earth’s distance from the sun creates a temperate environment in which water exists in all three of its states naturally.
A. Water’s Physical Properties
1. Unique Properties of Water
a. Hydrogen bonding: Water (H2O) sticks to things — it’s “wet.” Because the two hydrogen atoms (H2) and one oxygen atom (O) that make the molecule have slight electrical charges; the hydrogen atoms have aslight positive charge, while the oxygen atom has a slight negative charge. When several water molecules get together, the positive charge on one molecule is attracted to the negative charge on another, leading to a strong, electrical hydrogen bond.
As a result of this hydrogen bonding, and the “strength” that gives to water, water has:
i. surface tension: for instance, when you over-fill a glass, the water actually goes above the rim of the glass. Water has a very high surface tension. In other words, water is sticky and elastic, and tends to clump together in drops rather than spread out in a thin film.
Surface tension is what allows insects to walk on water, needles and paper clips (left) to float, etc. It allows you to “pull” water along a table top with your finger.
Surface tension is responsible for capillary action, which allows water (and its dissolved substances) to move through the roots of plants and through the tiny blood vessels in our bodies.
ii. Capillarity or capillary action: water tends to attract other water. So, for instance, in a partly filled glass, the glass “creeps” up the sides of the glass if the sides are already wet. Raindrops, running down a window “coalesce” to form larger drops. If you have a water drop on a table and “pull” another drop with your finger (through surface tension), the drop will actually “leap” to join the other drop once it is close enough. Thus water readily wets many materials. Capillary action allows a paper towel or a sponge to be used to soak up spilled water. Without this property, the nutrients needed by plants and trees would remain in the soil.
This polarity in water molecules is why water can dissolve so many substances. Pure water is very rare in nature because water easily dissolves many chemical compounds.
b. Heat Properties
Study Figure 7.2, 4CE p. 183 (7.5;3CE, p. 171), “Three states of water…”.
know:
- freezing/thawing are phase changes to solid from liquid and from solid to liquid
- condensation/evaporation are phase changes to liquid from gas and from liquid to gas
- sublimation refers to phase changes directly either from solid to gas or gas to solid (skipping the liquid stage)
* Other than “sublimation” (which may be unfamiliar to you), this should be straight forward.
- Water is the only natural substance that is found in all three states — liquid, solid (ice), and gas (steam) — at the temperatures normally found on Earth. Earth’s water is constantly interacting, changing, and in movement (the water cycle).
- Water freezes at 0 degrees on the Celsius scale; 100 degrees is water’s boiling point – at sea level (0 m) and normal air pressure (1013.5 mb). Water’s freezing and boiling points at sea level at normal air pressure are the baseline with which temperature is measured.
- Altitude makes a difference to the boiling point of water. In Edmonton, altitude 668 m, water actually boils at 98 C; in Lake Louise, AB, the highest community in Canada, 1534 m above sea level, water boils at 95 C! (The good news, when you’re mountain climbing at high altitudes and trying to boil water for tea is that you don’t actually have to get the water to 100 C to get it to boil!)
- Air pressure also makes a difference to the boiling point of water. At sea level, during periods of high air pressure (e.g. 1026 mb), water boils at 100.5 C. At sea level, during low pressure (e.g. 980 mb), water boils at 99 C.
- Water is unusual in that the solid form, ice, is less dense than the liquid form, which is why ice floats. Most other minerals and compounds, the solid state is denser than the liquid form.
- Water has a high specific heat index. This means that water can absorb a lot of heat before it begins to get hot. The high specific heat index of water also helps regulate the rate at which air changes temperature, which is why the temperature change between seasons is gradual rather than sudden, especially near the oceans.
i. Ice (Solid phase H2O)
As they cool, most compounds contract in volume. They increase in density so the same number of molecules now occupy a smaller volume. Water does this too, until about 4 degrees C. Below 4 C, water expands as hydrogen bonding causes ice to form hexagonal (six-sided) ice crystals. Thus, unlike most compounds, when water does solidify as ice it expands and decreases in density (ice is 0.91 times as dense as water) – it floats. And it occupies more space than liquid water. When water freezes in cracks in rock, as it expands it can actually shatter the rock (a weathering process called frost shattering); this is responsible for the talus (rock) slopes at the bottom of many cliffs in alpine areas.
On a global scale this is important because otherwise sea-ice and icebergs would sink! As it is, ice does float – barely! 86% of an iceberg/ice sheet is below water, only 14% above (just ask the Titanic).
Read Geo Report 7.1 (4CE, p.184; 3CE, p. 172), “Breaking roads and pipes.” Canadians, however, know all about “frost heaves” — water gets into cracks in the road; it freezes and expands, “heaving” the road into nasty bumps — and the damage they do to roads (and suspensions). This is also responsible for spring potholes on roads as well. If you leave water in a pipe or a hose and then allow that pipe/hose to freeze … again, the frozen water expands and can burst your pipes.
On a smaller scale it is important as a weathering process. When liquid water gets into a crack in rock and freezes it expands, breaking off pieces of rock. If you have taken the other course you will remember this is an important form of mechanical weathering, responsible for the talus slopes beneath cliffs in the Rocky Mountains).
Check out the Canadian Ice Services page and the U.S. Coast Guard International Ice Patrol.
ii. Water (Liquid phase H2O)
Water, unlike ice, easily assumes the shape of whatever container it’s in.
*Now follow along with Figure 7.5 (4CE, p. 185) / Figure 7.7 (3CE, p. 174), “Water’s heat-energy characteristics.” * This is important! The Figure in your text will really help!
We have mentioned earlier that latent heat is “hidden heat” and is associated with phase changes. Here we’ll unpack that a bit …
Heat energy has to be added to change ice to water.
80 calories of energy are needed to change 1 gram (g) of ice at 0°C to 1 gram (g) of water at 0°C.
Although energy is being added, the temperature of the water molecule does not change, only the phase changes (notice, the ice is at 0º C and the water is still at 0º C). This energy, then is not being used to heat the water (that would be sensible heat), just to change the phase from solid to liquid.
This energy, then is latent heat. Specifically, it is called the latent heat of melting. It is “hidden,” stored, or dormant in the water. We do not feel it as sensible heat (heat you can sense – you feel it as a temperature increase). But, if the process were reversed, when 1 g of liquid water freezes to become 1 g of ice, 80 calories of energy is released as sensible heat.
A further 100 calories of energy are needed to change 1 g of liquid water from 0°C to 100°C (boiling)
(NOTE: A “calorie” of energy is technically defined as the amount of energy needed to raise 1 g of liquid water by 1°C; thus 100 calories are needed to raise 1 g of liquid water by 100°C!
(You do NOT need to know this paragraph for the exam, but, for your information … calories in the sense we are talking about are related to, but should not be confused with FOOD Calories. For historical reasons, two main definitions of calorie exist. The small calorie or gram calorie (usually denoted “cal” with a lower case “c”) is the amount of heat needed to raise the temperature of one gram of water by one degree Celsius (as in this course). The large Calorie, food Calorie, or kilocalorie (Cal, Calorie or kcal) is most widely used in nutrition and is indicated as the nutritional energy value in (kilo or large) Calories per serving or per weight on food products. This is the amount of heat needed to cause the same increase in one kilogram of water. Thus, 1 kilocalorie (kcal) = 1000 calories (cal). Typically, in food science the large Calorie is commonly called Calorie (with a capital “C” to distinguish it from the smaller unit).
Thus a total of 180 calories of energy are needed to change 1 g of ice at 0ºC to 1 g of liquid water at 100°C (boiling):
80 calories: to take 1 g of ice at 0°C to 1 g of liquid water at 0°C
+ 100 calories: to take 1 g of liquid water at 0°C to 100°C
_________
180 calories
iii. Water vapour (Gas phase H2O)
Much more energy is needed to vaporize (evaporate) water into gas:
540 calories of energy are needed to change 1 g of liquid water at 100°C into 1 g of water vapour at 100ºC
Again, although energy is being added, the temperature of the water molecule does not change, only the phase changes (it stays at 100 degrees C). This energy, then is not being used to heat the water, just to change the phase.
This energy is called the latent heat of vaporization. Again, it is “hidden,” stored, or dormant in the water vapour. We do not feel it as sensible heat (able to be sensed as temperature increase). But, if the process were reversed, when 1 g of water vapour condenses to become 1 g of liquid water, 540 calories of energy is released as sensible heat!
Thus a total of 720 calories of energy are needed to change 1 g of ice (0ºC) to 1 g of water vapour (100ºC):
80 calories: to take 1 g of ice at 0°C to 1 g of liquid water at 0°C
+ 100 calories: to take 1 g of liquid water at 0°C to 100°C
+ 540 calories: to take 1 g of liquid water at 100°C to 1 g of water vapour at 100°C
_________
720 calories
iv. When H20 goes in the OPPOSITE direction – from water vapour (gas) to liquid, to solid (ice) – latent heat is released as sensible heat. When water vapour condenses into a liquid (for instance into a raindrop), 540 calories of energy is released as sensible heat (the latent heat of condensation). When water freezes into ice, 80 calories of heat is released as sensible heat (the latent heat of freezing).
v. In the real world …
In reality, in nature, liquid water is rarely already at 100°C (just jump into the Pacific or Atlantic Ocean and see). Thus, every 1 g of water evaporated from a stream, lake, soil, or leaf, etc. requires the absorption of more than 540 calories of energy for it to become water vapour. On average, approximately 585 calories of energy is required to evaporate 1 g of water from the real natural environment 9 (like a lake).
The same is true in reverse. When water vapour condenses, it rarely condenses at 100 degrees C. It condenses as water droplets in clouds, raindrops, fog, and dew. When this water vapour condenses as a water droplet in a cloud, a lot of energy is released. Typically closer to 585 calories is released. This is why summer thunderstorms and hurricanes – where condensation is occurring rapidly and in large amounts – are very powerful storms. Tremendous amounts of latent energy can be released within very short periods of time when water is being condensed quickly.
Water vapour is also an important greenhouse gas. As global temperatures rise, evaporation from lakes, rivers, and oceans increases, adding more water vapour in the atmosphere. A higher concentration of water vapour results in higher temperatures, resulting in more evaporation, etc., etc. This is an example of positive feedback: one change creates a cascading amplification of that change. More water vapour and latent energy in the atmosphere also can mean more instense, powerful storms and precipitation events.
(You don’t need to remember all the numbers, but do know the principles)
Look at the chart at the top of Figure 7.5 (4CE, p. 185) / Figure 7.7 (3CE, p.174), “Water’s heat-energy characteristics.”
B. Humidity
Humidity refers to the water vapour content in the air. In other words, humidity describes how much water vapour is there in the air. Some days the air seems really dry (low humidity). Other days, the air feels very damp (high humidity). Humidity is measured by relative humidity.
Relative humidity (RH) measures how much water vapour is actually in the air, compared with the amount of water vapour the air could potentially hold at that temperature.
Relative humidity =
(Actual water vapour content of the air / maximum amount of water vapour the air could hold at that temperature) x 100%
-
When the RH of air is 100%, the air is saturated. It can hold no more water at that temperature. At 100% RH, water vapour begins to condense to form clouds, fog, and precipitation. This is what the air is like when it’s raining, or really hot an humid in southern Ontario …
- When the RH of air is 50%, it feels pleasant – not too dry, not too wet. The air can still absorb more moisture through evaporation. This is what it’s like when you are on the coast or by a lake on a pleasant summer day …
-
When the air RH of air approaches 0%, it feels drier and drier – uncomfortably dry! Your lips chap. Your throat dries out. Your eyes hurt. This is one reason air on airplanes feels so uncomfortable! You are always thirsty … because the RH is usually very low. This is what it is like in hot, dry, desert areas, too.
An important principle in the discussion of relative humidity is: cooler air can hold less moisture than warmer air.
See Figure 7.7 (4CE, p. 187) / Figure 7.8 32CE, p. 176), “Water vapour content, capacity, and relative humidity.” Note that cooler air can be saturated with a small amount of moisture (the 5 a.m. circle). But warmer air could potentially hold much more moisture before it would be saturated (the 5 p.m. circle).
- Often you have early morning fog, but it “burns off” as the day heats up. The actual amount of moisture in the atmosphere is the same at dawn and mid afternoon, but the relative humidity drops as the air temperature increases. At dawn (cool temperature), the air is saturated, 100% RH – fog. Mid afternoon (warm temperatures) the air is no longer at 100% RH, it’s clear!
Thus, if you cool warm, moist air down, it may reach a temperature where it is saturated, 100% RH. At that temperature, condensation begins to occur. This is called the dew point temperature (because, in nature, this is the temperature at which dew – condensed water droplets – begins to form). Thus we can say that the temperature at which a given mass of air becomes saturated (and condensation begins to occur) is the dew point temperature.
For example, if you have a cold glass (lots of ice cubes!) on a hot day, condensation forms on the outside of the glass as water droplets. What you have done is you have cooled the air around the glass to the dew point temperature. Water vapour in the air around the glass condenses as water droplets. You have created dew! The same effect often happens naturally, when warm moist air moves over cold land, water, or ice surfaces. The air cools and water droplets form … as dew, fog, or frost. See Figure 7.8 (4CE, p. 187) / Figure 7.9 (3CE, p. 176), “Dew point temperature examples.”
To summarize: on a daily basis, if the amount of water vapour in the atmosphere stays the same:
-
- the RH is usually highest at night, because this the coolest time of the day (there is no insolation; the ground is radiating longwave energy). As the air cools, the RH rises. You are not adding any more moisture to the air. But cooler air can hold less moisture. Thus dew and fog tend to form just before dawn, when the ground and air are coldest, because the ground has radiated longwave radiation all night.
- The RH is usually lowest during the afternoon, the hottest time of the day (the ground is warmest, the air has heated to the warmest). Through the day, insolation heats the earth and the atmosphere. Dew disappears as the air temperature rises and the RH decreases (it re-vaporizes). Fog disappears (it re-vaporizes, too). Frost “melts” – either to liquid, or sublimates to vapour.
Don’t worry about “Expressions of relative humidity” or “Instruments for measuring humidity”
C. Atmospheric Stability
Air with fairly uniform specific temperature and humidity characteristics is called a “parcel” or an “adiabat.”
Warm air parcels or adiabats, tend to rise – they are buoyant (hot air rises). Cool air parcels tend to descend (cool air sinks).
Atmospheric stability refers to whether or not a parcel of air
- is going up (unstable), or
- staying put/descending (stable).
E.g. hot air balloons – a balloon with normal air in it will sit on the ground and go nowhere (stable). When the balloon’s burner is lit, the air inside the balloon is warmer than the air around the outside of the balloon – the balloon rises (unstable). As the air inside the balloon is allowed to cool, the balloon sinks (stable). See Figure 7.13 (4CE, p. 190) / Figure 7.16 (3CE, p. 179), “Principles of air stability and balloon launches.”
You may have noticed this in your home, too. The upstairs is usually warmer than the basement! Cool air sinks. Warm air rises. When my kids leave the back door open — right by the stairway going upstairs and the stairway going downstairs to the basement — the basement cools much more quickly! This is also why it is much more efficient to have hot air vents on the floor than in the ceiling. It also means that on a hot summer day, the basement will be a cooler place to be.
-
Lapse rates in non-moving (stable) air
Air cools as you increase in elevation because it gets thinner (remember Chapter 3!). Air higher up is less dense, thus cooler. This cooling with increasing altitude is expressed as a lapse rate.
In stable, non-rising air, the normal lapse rate is 6.4-6.5°C/1000m. That means that if you went up a mountain 1000 m high. the air would be 6.4-6.5°C cooler at the top. The opposite is also true: if you descend a 1000 m mountain, it will be 6.4°C warmer at the bottom. This is a theoretical value, based only upon the changes in atmospheric density with altitude. Note that the normal lapse rate only applies in non-rising, stable air!
In actual natural environments, air may cool at a rate slightly different than the normal lapse rate because of local conditions – albedo, winds, etc. This actual, real world lapse rate at a given location is called the environmental lapse rate. It will vary from place-to-place and from day-to-day given local conditions. Again, note that the environmental lapse rate only applies in non-rising, stable air!
It is because of these lapse rates that the air is cooler at the top of a mountain (even to the point of having ice/snow all year round) rather than at the base. It is also a reason why, in winter, Vancouverites can ski on Grouse Mountain (below 0ºC), right beside the city, at sea-level, which can be comfortably above 0ºC).
-
Lapse rates in moving (unstable) air
Unstable, rising air parcels (adiabats) cool at different rates, depending on the moisture conditions in the rising air. Note, this refers to rising, unstable air:
a. Dry Adiabatic Rate (DAR)
Rising air, as long as air has not yet cooled to the dew point temperature (so no condensation is yet occurring), cools at an average rate of 10°C/1000m. When the air is rising, if you were to climb the 1000 m mountain during a hot afternoon when air is rising, you would expect the top to be 10°C cooler than the base. Or, if you were to descend the mountain, the base would be 10°C cooler than the summit.
This is the dry adiabatic rate (DAR). Note, the DAR only applies in rising, unstable air! And, only when the rising, unstable air is still warmer than the dew point temperature.
See “Geosystems in Action 7: Adiabatic cooling and heating”, p. 192
b. Moist Adiabatic Rate (MAR)
Eventually a rising, unstable air parcel (adiabat) may cool to its dew point temperature (remember, the air is cooling at 10°C as it rises, and cooler air can hold less water vapour). Eventually the air may cool to dew point temperature. At the dew point temperature, the air parcel is at 100% RH. Condensation begins to occur. This takes the form of water droplets forming in the atmosphere – clouds!
As this condensation occurs, the latent heat of condensation (540 calories per 1 g) is released. This release of heat energy reduces the lapse rate.
The average moist adiabatic rate is 6°C/1000m. Note, the DAR only applies in rising, unstable air! And, only when the rising, unstable air is at or below the dew point temperature!
So … imagine the air at sea level is 20ºC. The air is rising, being pushed up and over a mountain. We’ll say that the dew point temperature (the temperature at which condensation would occur [RH would be 100%]) is 0ºC (remember, no moisture is being added, but cooler air can hold less water than warmer air).
- at 1000 m above sea level, the air temperature will be 10ºC (it drops at the DAR because it is above the dew point temperature)
- at 2000 m above sea level, the air temperature will be 0ºC (it drops at the DAR because it is above the dew point temperature)
- above 2000 m, the air temperature will drop at the MAR, because it is now below the dew point temperature of 0ºC
- at 2000 m clouds will also begin to form because this is where condensation is occurring
- at 3000 m above sea level, the air temperature will be -6ºC (it drops at the MAR because it is below the dew point temperature)
Don’t worry about the section entitled “Stable and Unstable Atmospheric Conditions,” 4CE, pp.191, 193-194; 3CE, pp. 181, or Figure 7.14 (4CE, p. 193) / Figure 7.19 (3CE, p. 182), “Stability – three examples.” They will confuse you!
II. Clouds and Fog
A. Clouds
Clouds are aggregations of tiny moisture droplets and ice crystals that are suspended in air, great enough in volume and concentration to be visible. (Fog is simply a cloud in contact with the ground).
-
Cloud formation
As air rises and may eventually reach the dew point temperature – relative humidity of 100%. The air is saturated. At that temperature, it cannot hold any more water vapour (renumber cold air can hold less water vapour than warm air).
At this level, water vapour condenses around cloud-condensation nuclei, microscopic particles of dust, soot, ash, salt from sea spray, etc. (from volcanoes, forest fires, dust storms, human pollution, etc.). Water droplets have to condense around solid particles. In nature there is always plenty of natural or human-produced pollution to provide more than enough condensation nuclei. In pure air, condensation cannot occur.
NOTE: this is why your car always looks dirty after a rainfall! Every one of those raindrops that landed on your car – and then evaporated when the sun came out – had a solid particle in it. The water evaporates away. The condensation nuclei are left behind on your nicely washed car.
Most raindrops are formed by a collision-coalescence process.
- As unstable air rises, the first tiny drops condense when the dew point temperature is reached.
- As these droplets continue to move upward in a cloud (the unstable air keeps rising after the dew point temperature is reached, cooling at the MAR) they collide with others and coalesce to form a larger drop.
- The droplet will only fall when its mass is big enough (combined with force of gravity) to overcome the upward movement of the unstable air.
- As it falls, it will collide with other droplets, forming bigger and bigger drops.
- When the drops are too heavy for the updrafts to keep aloft, they fall as raindrops.
See Figure 7.16 (4CE, p. 194) / Figure 7.20 (3CE, p. 183), “Moisture droplets and raindrops.”
In my (Prairie) experience, this occurs quite often. Through hot, summer days the land heats and air begins to rise. By afternoon, strong updrafts have developed, resulting in lots of rising, unstable air. Towering clouds (thunderheads) develop as the strong updrafts push the warm, moist air higher and higher. Only when drops become very large are they able to overcome the strong updrafts of the unstable air. So when the rain falls it falls as large raindrops.
At the coasts, most precipitation results associated with weak updrafts associated with a warm front. Weak updrafts mean the drops may be quite small when they overcome the lifting force of the updraft … resulting in drizzle. Drizzle-sized raindrops could not fall during Prairie storms because they would never be heavy enough to overcome the force of the strong updrafts.
In cold clouds, the Bergeron ice-crystal process explains how ice crystals add water vapour to form larger water or ice droplets. In a very cold, or supercooled cloud, there are both liquid droplets and also ice crystals. Water vapor molecules “prefer” to coalesce with — or deposit onto — ice crystals rather than to condense into a liquid droplet. Once water vapor begins to deposit onto the ice crystal there are two results: the ice crystals grow and cloud water droplets get fewer and smaller. When the ice crystal leaves the cloud it is called a snowflake. Next snowflake you see, you can say, “Hah! You product of the Bergeron ice-crystal process, you!”
- See Figure 7.17 4CE p. 195 (7.21 3CE, p. 184-185), “Principal clouds.”
- World Meteorological Organization’s Cloud Atlas. The definitive resource on all things “cloud.”
Clouds are classified by altitude and shape. There are four main groups of clouds: high, middle, low, and vertically-developed. Clouds can easily be identified by knowing these five terms:
- cirrus – very high, ice crystals, all white, very thin
- alto – middle, ice and/or liquid, white and grey, thin
- cumulus – puffy appearance, all vertically-developed clouds begin with “cumu-“
- nimbus – cloud must be precipitating
- stratus – cloud is spread out, most likely covers the sky
Clouds that are developed horizontally (flat layers) are called stratiform clouds. These clouds are typically associated with warm fronts:
a. high level cirrostratus appear as a thin “veil” of ice crystals
b. mid-level altostratus appear a high, light grey sheet across the sky.
c. low level stratus clouds are like dull, grey blanket close to the surface (east and west coast people know all about these!) — when it is actually precipitating, they are called nimbostratus
d. cirrocumulus (high level) clouds, like other cumulus clouds, appear as layers of “cotton balls.” They can appear as a uniform pattern, patchy rows, wave patterns, etc. They form as daytime warming causes land to heat, air to rise, and condensation to occur.
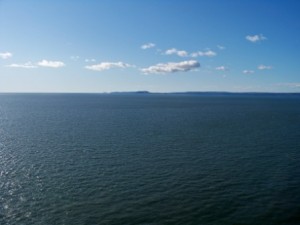
Altocumulus clouds, Weymouth, UK
e. altocumulus (mid level) cumulus clouds are similar “cotton balls” but lower in altitude
f. stratocumulus (low-level) clouds are low, heavy, dark storm clouds
g. When cumulus clouds become large (due to much evaporation and strong updrafts), cumulonimbus clouds (thunderheads) can develop, causing heavy rain, hail, thunder, and/or lightning. See Figure 7.18 4CE, p. 196 (7.22 3CE, p. 187), “Cumulonimbus thunderhead.”
Find these clouds on Figure 7.17 (4CE)/7.21 (3CE) ”Principal clouds”!
h. Wispy, high altitude clouds of ice crystals are simply called cirrus clouds. These cirrus clouds are higher and less uniform than cirrostratus clouds. The appear as isolated wisps of white, very high in the atmosphere. See “Cirrus” on Figure 7.17 (4CE)/7.21 (3CE), “Principal clouds”!
B. Fog
Fog is a cloud layer on the ground, with visibility of less than 1 km.
Because condensation is occurring, fog implies the temperature at the ground is at, or below, the dew point temperature of the air. Fog is usually capped by a layer of warmer air, preventing the air from rising (a temperature inversion).
- Advection fog forms when air moves horizontally from one place to another. Warm, moist air may move over colder water currents. When this happens, the air cools and fog results. For instance, on the coast, warm, maritime tropical air may move north over cooler waters, causing thick fog to develop. This is typical off both coasts as warm air masses move north, pushed by south-westerly winds (from the south-west to the north east). See Figure 7.21 4CE, p. 198 (7.23 3CE, p. 188).
- Upslope Fog forms when air is moving, forced to rise to go over a mountain, it may cool (DAR) to the dew point temperature, causing fog to form when the dew point is reached. This is common in mountain regions. This is why tall mountains often have clouds or fog around their tops. The cold surface (glaciers) and lower air temperatures at high altitudes cool the rising air to the dew point.
-
Valley Fog forms during the night. Valley winds may move moist air down into cold valley bottoms (remember katabatic winds). This often happens in river valleys. See Figure 7.22 4CE, p. 198 (7.26 3CE, p. 189), “Valley Fog.”
- Radiation or evaporation fog forms when there is no air movement.
A surface cools as it radiates energy to the point where the overlying air reaches the dew point. This typically happens on cool nights. Land radiates longwave energy all night long (the land is coolest right before dawn). The air above it cools. Often fog develops just before dawn when the air reaches its dew point temperature, and “burns off” as the land heats from the solar radiation, during the morning. See Figure 7.19 4CE, p. 195 (7.27 3CE, p. 189), “Radiation Fog.”
- Steam Fog
Steam fog occurs when cooler air rests above warmer water and vapor that evaporates into the air cools to its dew point. This can occur over hot springs. Or it can occur in the Arctic when icy cP air moves over open water (which is relatively warm compared to the air). This is sometimes called Arctic sea smoke.
- Ice-crystal fog is relatively common in extremely cold climates, where any moisture in the air freezes into ice crystals. Figure 7.20 4CE, p. 197 (3CE, Figure 7.28, p. 189).
Fog is primarily associated with coastal areas (Figure 7.24, p. 199). A combination of relatively warm coastal waters and cooler land creates ideal conditions for fog. Fog is much less common in drier continental areas. The foggiest place in Canada? Newfoundland’s Avalon Peninsula – upwards of 200 days of fog per year.
The importance of fog as a source of moisture for Earth ecosystems (even human drinking water) is summarized in “Geosystems Now: Getting water from air in arid climates” (beginning of Chapter 7 in your text, p. 181) and in a Scientific American article, “Fog for a Thirsty Planet”
III. Precipitation
A. Dew, Fog-Drip, Rime
These are the smallest forms of precipitation.
Dew forms as tiny water droplets on cold surfaces at night when the air reaches the dew point temperature. Most is evaporated when the sun rises.
Fog-drip occurs when low clouds or fog move through vegetation and deposit small water droplets on leaves. This is particularly important where forests extend into persistent cloud sheets (e.g. Hawaii, parts of California).
Rime is formed when fog or dew droplets freeze onto vegetation. In winter the trees are often covered in white ice crystals. This is called rime.
B. Rain
Rainfall consists of liquid water droplets. Droplet size is largely a function of the strength of the upward movement of air. Strong updrafts (producing thunderheads), result in larger drops because the drops have to enough mass to overcome the updrafts. Less strong updrafts (associated with stratiform clouds) tend to produce smaller drops.
The size of droplets also affects the velocity with which they hit the ground (which determines how much erosion the rain causes). In still air, a small drop of 0.2mm in diameter falls at a rate of 70 cm/s; a large drop of 2 mm falls at a rate of about 650 cm/s. The larger drop will cause more erosion! And it will hurt more if it hits you!
Rain is measured simply by bucket-shaped gauges that fill with water to show the amount that has fallen.
C. Snow
On a global basis, probably less than 1% of total annual precipitation falls as snow. But in Canada and parts of the United States, a much higher percentage of our precipitation falls this way. Click here for the current snow report for Canada (in season).
On average, 12 cm snow, when melted, is equivalent to 1 cm of rain water. However not all snow contains the same water equivalent. There is “dry” snow – typical of the Prairies. And there is “wet” snow – typical of coastal areas. Very cold, dry air snow contains less moisture; it may take as much as 1 metre of snow to yield 1 cm of water.
In much of Canada, seasonal snowmelt is an important source of moisture for vegetation and plant life. This meltwater is stored in lakes, rivers and groundwater, replenishing the system. It is also the source for evaporation and summer thundershowers.
Snow also insulates the vegetation and soil, preventing deep frost penetration.
Snowflakes (of course) vary considerably in size and shape. In general, near freezing conditions allow large flakes to form, which fall relatively quickly. Extreme cold conditions allow only small, slow flakes. Because snowflakes are light, their distribution is very much affected by local topography and winds.
Snow does not necessarily directly recharge regional water supplies. Water may stay frozen for months, years, even centuries (in ice caps) before being released.
Measuring snowfall is never accurate. In Canada and the U.S. buckets (as for rainfall) are used, and a water equivalent can be estimated. But snow blows so much that the buckets themselves often cause localized air currents and underestimate actual snowfall. Other attempts to measure it use long poles to measure depth, but depth inevitably varies with topography and winds!
D. Hail
Hail is formed when updrafts in thunderclouds carry raindrops upward into extremely cold areas of the atmosphere, where they freeze and merge into lumps of ice. When the lumps become too heavy to be supported by the updraft, they fall to the ground at speeds of up to 100 km/h or more.
Hailstones as large as grapefruit have been reported in Canada, but even smaller hail can be dangerous and can cause extensive damage in a matter of minutes. Canada — especially southern Alberta (Calgary) has had some of the costliest hailstorms ever: http://en.wikipedia.org/wiki/List_of_costly_or_deadly_hailstorms
Hail tends to be very localized, so data on its frequency and duration is scarce. Often one corner of a field will be destroyed, but the rest of a field will be untouched.
The amount of water it actually contributes to a region is negligible. Because it is hard to measure and doesn’t amount to much quantity, hail is normally measured in a region as simply “number of days with hail per year.”
Other types of precipitation are
You do not need to know these for an exam, but these websites are good resources for further study. Other good references detail the dangers to people of ice storms (again, for your edification, not for an exam),
- A large ice storm did considerable damage to Atlantic Canada and the NE United States in December 2013.
Worth reflecting on …
- American Baptist churches urge their members to:
- Affirm the goodness and duty of God’s creation.
- Acknowledge our responsibility for stewardship of the Creator’s good Earth.
- Learn of the environmental dangers facing the planet.
- Recognize that our practices and styles of life has had an effect on the environment.
- Pursue a lifestyle that is why is and responsible in light of our understanding of the problems.
- Exert our influence in shaping public policy and insisting that industries, businesses, farmers, and consumers relate to the environment in ways that are sensible, healthy, and protective of its integrity.
- Demonstrate concern with “the hope that is within us,” as despair and apathy surround us in the world [Romans 12:21].
- Become involved in organizations and actions to protect and restore the environment and the people in our communities.
How do you feel about that? Do you agree? Feel free to discuss this quote on the course discussion site …
2. When Rosalind Picard was younger, she assumed religion was for emotionally or intellectually stunted people. The experience of reading the Bible for herself challenged all her previous assumptions (she is a professor of affective computing at MIT). Here are three short videos sharing her testimony and Christian journey. A fascinating insight into the spiritual life of a leading scientist:
- Rosalind Picard – personal story [part 1] – Coming to faith – YouTube
- Rosalind Picard – Personal story [part 2] – Do you ever face doubts? – YouTube
- What are the strongest grounds for rejecting Christian faith? – YouTube
To review …
Check out the resources at masteringgeography.com
This page is the intellectual property of the author, Bruce Martin, and is copyrighted © by Bruce Martin. This page may be copied or printed only for educational purposes by students registered in courses taught by Dr. Bruce Martin. Any other use constitutes a criminal offence.
Scripture quotations marked (NLT) are taken from the Holy Bible, New Living Translation, copyright © 1996. Used by permission of Tyndale House Publishers, Inc., Wheaton, Illinois 60189. All rights reserved